YANG'S GROUP
in NUS, Singapore
About Our Research
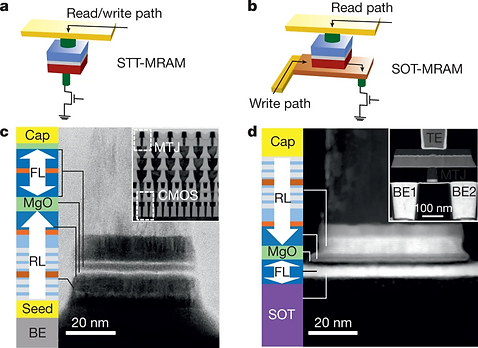
Fig 1. Magnetic random access memory.
Source: Yang, H. et al., Nature 606, 663–673 (2022)
Selected publications
Yang, H. et al., Nature 606, 663 (2022)
Kim, S. et al., Nat. Mater. 21, 24 (2022)
Chen, X. et al., Nat. Mater. 20, 800 (2021)
Mishra, R. et al., Phys. Rev. Applied 15, 024063 (2021)
Cai, K. et al., Nat. Electron. 3, 37 (2020)
Spin-orbit torque (SOT) MRAM
Spin-orbit torque MRAM is a type of magnetic random-access memory that leverages the spin-orbit torque (SOT) to switch the magnetization direction of a magnetic tunnel junction (MTJ) and store information. In contrast to spin-transfer torque (STT) MRAM, which uses a current passing through the tunnel barrier to flip the MTJ's magnetization, SOT-MRAM generates spin currents by passing an electric current through a spin source layer (Fig. 1). These currents interact with the MTJ's magnetic layer and exert a torque on its magnetization, allowing for the direction of magnetization to be flipped. Unlike STT-MRAM, SOT-MRAM prevents write errors during read operation and reduces a voltage breakdown, enabling it to offer superior speed, endurance, and energy efficiency. We are investigating various materials including ferrimagnets and antiferromagnets as well as device structures to improve the switching efficiency and operation speed of SOT-MRAM.
Energy harvesting
The field of energy harvesting is evolving rapidly, and spin nano-oscillators or non-linear Hall effect are emerging as promising technologies that utilize spintronic or magneto-electric effects to extract energy from the environment. One such approach involves the use of spin Hall nano-oscillators, which utilize the oscillation of magnetic elements in a magnetic tunnel junction to extract energy from the environment, such as WiFi signals. By connecting multiple oscillators in a topological manner, we can obtain high rectified voltage and demonstrate a practical wireless energy-harvesting system, as depicted in Fig. 2, capable of powering an LED.
Another promising technology involves the non-linear Hall effect (NLHE), which enables the conversion of microwave energy into usable electrical voltage. Our recent studies have shown that topological materials, such as TaIrTe4, exhibit room-temperature NLHE, enabling the wireless radiofrequency (RF) rectification with zero external bias and magnetic field, as demonstrated in Fig. 3.
These innovative energy-harvesting technologies have the potential to revolutionize power generation and reduce our reliance on traditional sources of energy. By harnessing the energy surrounding us, we can make significant progress towards sustainable and clean energy for the Internet of Things (IoT).
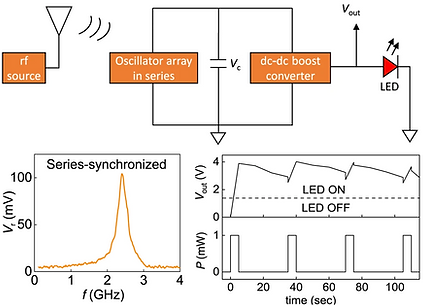
Fig 2. Voltage rectification with eight synchronized oscillators which can light up the LED.
Source: Sharma, R. et al., Nat. Commun. 12, 2924 (2021)

Fig 3. Rectification demonstration based on the nonlinear Hall effect in TaIrTe4.
Source: Kumar, D. et al., Nat. Nanotech. 16, 421–425 (2021)
Selected publications
Sharma, R. et al., Nat. Commun. 12, 2924 (2021)
Kumar, D. et al., Nat. Nanotech. 16, 421–425 (2021)
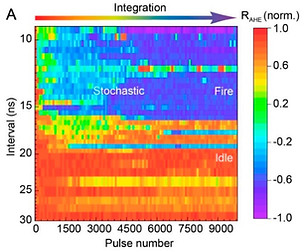
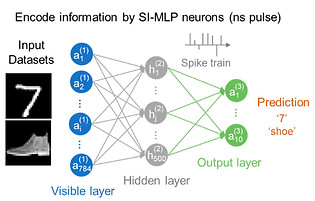
Fig 4. (left) Stochastic integration and fire behaviors in a SOT device excited by nanosecond pulses. (right) Network structure of the “stochastic integration” MLP classification model.
Source: Yang, Q. et al., Nano Lett. 22, 8437 (2022)
Spin-based unconventional computing
In recent times, the demand for computing systems that can efficiently process vast amounts of data generated by modern technology has grown significantly. However, traditional von Neumann computing architectures are limited in terms of both computational power and energy efficiency. As a result, new computing paradigms have emerged, including Ising and neuromorphic computing.
Spin-based computing has become increasingly popular due to its energy-efficient and scalable nature. Spintronics has demonstrated significant potential in both Ising and neuromorphic computing. Ising computing uses the Ising model from statistical physics to solve optimization problems, while neuromorphic computing takes inspiration from the structure and function of the human brain.
Spintronics devices that utilize spin transfer torque (STT) and spin orbit torque (SOT) have been proposed as potential hardware solutions for both Ising and neuromorphic computing, as depicted in Fig. 4. The utilization of such devices can enable unconventional computing systems with unique and exciting features.
Selected publications
Yang, Q. et al., Nano Lett. 22, 8437 (2022)
Mishra. R. et al., Phys. Rev. Applied 11, 054065 (2019)
Mishra. R. et al., Nat. Commun. 10, 248 (2019)
Terahertz spintronics and magnon torques
The electrical spin torque, which involves moving charges, has inherent limitations such as Joule heat and corresponding power dissipation, as well as a short spin diffusion length. To overcome these fundamental obstacles, researchers have turned to magnon torque, where angular momentum is carried by precessing spins. Terahertz (0.1–10 THz) spintronic technologies have been extensively researched for their wide range of promising applications, including the observation of magnon-mediated angular momentum flow in antiferromagnetic materials. In recent studies, we have demonstrated magnon-torque-driven full magnetization switching at room temperature. Utilizing ultrafast THz excitation and detection probe techniques, we discovered that magnons propagate in NiO (<50 nm) at superluminal velocities of up to 650 km/s as shown in Fig. 5. This breakthrough has significant implications for the development and design of next-generation THz high-speed, energy-efficient, low-dissipation spintronic devices, thereby promoting the 6G era.
Selected publications
Shi, G. et al., Phys. Rev. Applied. 19, 034039 (2023)
Cheng, L. et al., Sci. Adv. 9, eadd7856 (2023)
Lee, K. et al., Nat. Nanotechnol. 16, 1337–1341 (2021)
Wang, Y. et al., Science 366, 1125–1128 (2019).
Cheng, L. et al., Nat. Phys. 15, 347 (2019)
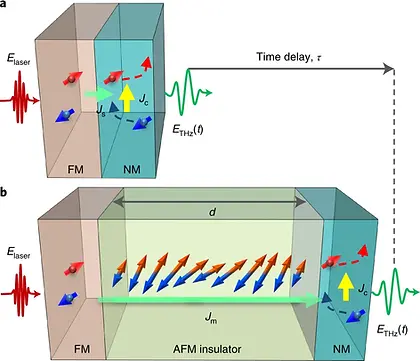
Fig 5. Schematics of magnon-mediated spin transport in an insulating antiferromagnetic material.
Source: Lee, K. et al., Nat. Nanotechnol. 16, 1337–1341 (2021)
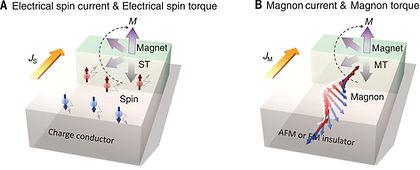
Fig 6. Two types of spin-angular-momentum-transfer torque.
Source: Wang, Y. et al., Science 366, 1125–1128 (2019).

Fig. 7. hBN-encapsulated graphene with broken inversion symmetry and nonlinear transport property..
Source: He, P. et al., Nat. Nanotechnol. 17, 378 (2022)
Two-dimensional van der Waals materials and heterostructures
Two-dimensional materials possess desirable characteristics for spintronics, including strong spin-orbit coupling, extended spin relaxation time, and reduced global symmetries. Specifically, materials with low crystal symmetry have the potential to produce out-of-plane damping-like spin-orbit torques, which could enable deterministic and energy-efficient switching of perpendicular magnets. Moreover, integrating two-dimensional van der Waals materials into heterostructures allows for the amalgamation of the properties of the constituent materials and the generation of novel electronic phases at their interfaces. This presents an opportunity to design artificial structures with predetermined characteristics. Therefore, two-dimensional materials and their related heterostructures hold great promise as leading candidates for next-generation spintronic devices.